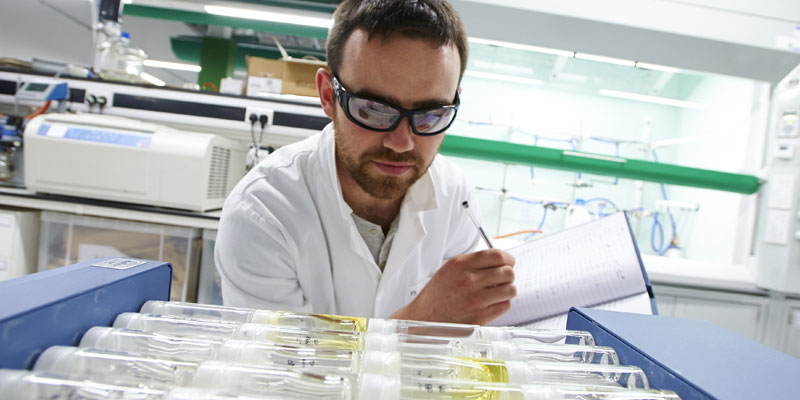
Green Synthesis
The GCCE has long played a role in devising tools and guides for synthetic organic chemists, including spearheading the influential CHEM21 solvent guide and metrics toolkit.
We are continuing this tradition by undertaking research to design and develop more benign reaction protocols for common synthetic transformations, with a particular current focus on halogenation.
We champion the synthesis of bio-derived platform molecules (building-block chemicals from biomass), and their conversion to an array of sustainable products such as monomers and polymers, solvents, surfactants, anti-oxidants and chelators.
Our expertise in developing cleaner synthetic routes and processes makes the production of a range of chemicals possible in a manner which maximises efficiency and minimises waste. We have considerable experience in the development and use of key enabling technologies such as catalysis, flow reactors, bio-based solvents, sonochemistry and microwaves in synthesis, all of which can enhance processing and are considered as cornerstones of green synthesis.
Our diverse expertise supports the development of biorefineries, both by demonstrating the valuable products that can be produced sustainably and providing the key enabling technologies to bring about the required synthesise. We also have a particular focus on the Circular Economy, especially around our plastics and other polymers.
Contact us
Green Chemistry Centre of Excellence
greenchemistry
+44 (0)1904 322567
Department of Chemistry,
University of York,
Heslington,
York YO10 5DD,
United Kingdom.
@@GreenChemYork
Facebook
We undertake research into both the synthesis and use of bio-based platform molecules, these are defined as:
“…a bio-based (or bio-derived) chemical compound whose constituent elements originate wholly from biomass (material of biological origin, excluding fossil carbon sources), and that can be utilised as a building block for the production of other chemicals.” [1]
BioPMs are typically small, relatively simple molecules of low value and ideally high production volumes (or at least the potential to be produced on scale), and are seen as the bio-based equivalent to crude-oil derived base chemicals (e.g. ethane, propene and benzene).
BioPMs typically contain significantly more heteroatom content than traditional crude-oil derived base chemicals, and therefore most of our research focuses on maximising the use of this inherent increased chemical functionality (fig 1). We developed the BioLogicTool concept as means of visually assessing the use of this high heteroatom content (fig 2), a free Excel tool is available from the publisher.[2] We were also involved in the development of the UKBioChem10, a list of the top platform molecules that industry and academia in the UK should focus research effort towards.[3]
Figure 1: Non-renewable base chemicals vs. bio-based platform molecules.[2]
Figure 2: BioLogicTool plots to aid visualisation of heteroatom content of bioPMs and routes through to products.[2]
We have prepared a range of key derivative chemicals from platform molecules, these being precursors to products that include solvents, monomers, polymers, surfactants, antioxidants, metal chelators and catalysts (fig 3).[4-12] Recent highlights include the synthesis of fully bio-based terephthalate via the Diels-Alder addition of ethene with furandicarboxylic acid diethyl ester (fig 4)[4]; decarboxylation of the amino acids lysine [5] and glutamic acid [6] for preparation of monomers; the application of electrochemistry (in collaboration with UC Davis, US) on levulinic acid to prepare novel secondary alcohol diols [7] and the modification of a wheat straw wax derived β-diketone for enhanced metal chelation in biphasic systems.[8]
Figure 3: Uses of platform molecules
Figure 4: Our new route (C) to biobased terephthalate via the Diels-Additions and dehydration of ethene with FDEE.
Relevant Publications
- Chapter 4: Platform Molecules, Farmer, T. J. & Mascal, M., in Introduction to Chemicals from Biomass. Clark, J. H. & Deswarte, F. (eds.). 2 ed. John Wiley & Sons, 2014, 89-155
- BioLogicTool: A Simple Visual Tool for Assisting in the Logical Selection of Pathways from Biomass to Products, Lie, Y., Ortiz, P., Vendamme, R., Vanbroekhoven, K. & Farmer, T. J., Ind. Eng. Chem. Res. 2019, 58 (35), 15945–15957
- http://ukbiochem10.co.uk/
- Synthesis of Biobased Diethyl Terephthalate via Diels–Alder Addition of Ethylene to 2,5-Furandicarboxylic Acid Diethyl Ester: An Alternative Route to 100% Biobased Poly(ethylene terephthalate), Ogunjobi, J.K, Farmer, T.J., McElroy, C.R., Breeden, S.W., Macquarrie, D.J., Thornthwaite, D. & Clark, J.H., ACS Sustainable Chem. Eng. 2019, 7, 9, 8183–8194
- Renewable Self-Blowing Non-isocyanate Polyurethane Foams from Lysine and Sorbitol, Clark, J. H., Farmer, T. J., Ingram, I. D. V., Lie, Y. & North, M. Eur. J. Org. Chem., 2018, 31, 4265-4271
- Facile and rapid decarboxylation of glutamic acid to γ-aminobutyric acid via microwave-assisted reaction: Towards valorisation of waste gluten, J. Clean. Prod. 2018, 205, 1102-1113
- Electrochemical Coupling of Biomass-derived Acids: New C8 Platforms for Renewable Polymers and Fuels, Wu, L., Mascal, M., Farmer, T. J., Perocheau Arnaud, S. & Chang, M. A. W., ChemSusChem, 2017, 10, 166–17, Open Access
- Modification of bio-based β-diketone from wheat straw wax: synthesis of polydentate lipophilic super-chelators for enhanced metal recovery, RSC Adv., 2019, 9, 3542-3549, Open Access
- Dihydrolevoglucosenone (Cyrene) as a bio-based alternative for dipolar aprotic solvents, Sherwood, J., De Bruyn, M., Constantinou, A., Moity, L., McElroy, R., Farmer, T. J., Duncan, T., Raverty, W., Hunt, A. J. & Clark, J. H., Chemical Communications, 2014, 50, 9650-9652
- Rapid and efficient biphasic liquid extraction of metals with bio- derived lipophilic β-diketone, Hunt, A. J., Farmer, T. J., Asemave, K., Byrne, F. P. & Clark, J. H., RSC Advances, 2016, 6, p. 95789-95792
- Ring opening metathesis polymerisation of a new bio-derived monomer from itaconic anhydride and furfuryl alcohol, Bai, Y., De Bruyn, M., Clark, J. H., Dodson, J. R., Farmer, T. J., Honoré, M., Ingram, I. D. V., Naguib, M., Whitwood, A. C. & North, M., Green Chemistry, 2016, 18, 3945-3948, Open Access
- p-Cymenesulphonyl Chloride: A Bio-based Activating Group and Protecting Group for Greener Organic Synthesis, Farmer, T. J., Clark, J. H., Gothe, M. L., Macquarrie, D. J., Sherwood, J., J. Braz. Chem. Soc., 2015, 26, 1914-1919, Open Access
Catalysis, and its associated energy saving and improved product selectivity, represents one of the key technologies in greener synthesis.
Facile recovery and reuse of heterogeneous catalysts means that their application can often reduce the environmental damage caused by traditional chemical processes and reactions. We are active in the development of new heterogeneous catalysts, in applying them in reactions involving platform molecules, and in their characterisation via a range of available analytical tools (N2 porosimetry, solid-state NMR, SEM, TEM etc.).
In collaboration with Professor Mark Mascal (UC Davis) we have shown that a porous lignin-derived solid support can be easily recovered during the biorefinery process for converting polysaccharides in lignocellulose into CMF. This recovered solid support can have its textural and polar properties fine-tuned through thermal treatment and can be easily sulfonated to produce a reusable solid acid catalyst (fig 1).[1]
Figure 1: CMF-lignin: a novel mesoporous heterogeneous catalyst direct from a biorefinery process
We have previously demonstrated that simply lab-grade K60 silica can be thermally treated and result in an effective catalyst for the direct formation of amides from aniline and a range of carboxylic acids.[2] This was further extended to show that mesoporous silicas reduce the overall catalyst loading required and also that the system operates effectively in flow.[3] Recently we have demonstrated how computational modelling allows for prediction of reagent solubility and product precipitation, meaning a recirculating system is effective and that high boiling point (and therefore lower voc releasing) solvents such as p-cymene can be used (fig 2).[4]
Figure 2: Computational guidance to enhance catalyst performance in a continuous recirculating amidation
Depending on the complexity of the catalyst, it can take many steps to synthesise some of the more complex ligands often used for metal centred catalysis and inorganic catalysts tend to require intense purification to remove contaminants. This considered, we investigated the direct, non-processed use of dried seaweed as a catalyst for the cycloaddition of CO2 to epoxides for the synthesis of cyclic carbonates (fig. 3). Kelp was initially chosen for investigation due to the naturally high concentration of halogens (iodide in particular) and Group 1 and 2 metals.[5]
Figure 3: Catalysts from seaweed for use in the synthesis of cyclic carbonates.
We have a particular interest in the use of biocatalyst both for the synthesis and breakdown of polymers (fig. 4). Several of the highly functional and reactive monomers we prepare from biomass require the milder polymerisation conditions only achieved through the use of enzyme catalysts.[6-11] Work has included the immobilisation of enzymes for use in polycondensations.[12]
We have also used certain enzymes to mimic biodegradation conditions in the environment, thus allowing us to assess the bio-circularity of both current and new innovative polymers.[13] The CSTP also studied the negative effects of microwave irradiation on the efficacy of lipases.[14]
Figure 4: Enzymatic Circle for the Synthesis, Functionalization, Modification, and Hydrolysis of Bio-Based Polyesters. (Figure from: Pellis et al. 2016, Trends Biotechnol., 34, 316-328)
Relevant Publications
- Processed Lignin as a Byproduct of the Generation of 5-(Chloromethyl)furfural from Biomass: A Promising New Mesoporous Material, Budarin, V. L., Clark, J. H., Henschen, J., Farmer, T. J., Macquarrie, D. J., Mascal, M., Nagaraja, G. K. & Petchey, T. H. M., ChemSusChem, 2015, 8, 4172-4179.
- Clean, reusable and low cost heterogeneous catalyst for amide synthesis, Comerford, J. W., Clark, J. H., Macquarrie, D. J., & Breeden, S. W., ChemComm, 2009, 2562-2564.
- Mesoporous Structured Silica – An improved catalyst for direct amide synthesis and its application to continuous flow processing, Farmer, T. J., MacQuarrie, D. J., Clark, J. H., Breeden, S. W., & Comerford, J. W., ARKIVOC, 2012, (vii), 282-293.
- Optimization of Amidation Reactions Using Predictive Tools for the Replacement of Regulated Solvents with Safer Bio-based Alternatives, Petchey, T.H.M., Comerford, J.W., Farmer, T.J., Macquarrie, D.J., Sherwood, J. & Clark, J.H., ACS Sustainable Chemistry & Engineering, 2017, 6, 1550-1554.
- Laminaria Digitata and Palmaria Palmata Seaweeds as Natural Source of Catalysts for the Cycloaddition of CO₂ to Epoxides, Comerford, J.W., Gray, T., Lie, Y., Macquarrie, D.J., North, M. & Pellis, A., Molecules 2019, 24(2), 269, Open Access
- Work‐hardening Photopolymer from Renewable Photoactive 3,3’‐(2,5‐Furandiyl)bisacrylic Acid, Lie, Y., Pellis, A., Funes-Ardoiz, I., Sampedro, D., Macquarrie, D.J. & Farmer, T.J., ChemSusChem 2020, 13, 4140-4150, Open Access
- Sustainable Galactarate‐Based Polymers: Multi‐Enzymatic Production of Pectin‐Derived Polyesters, Vastano, M., Pellis, A., Botelho Machado, C., Simister, R., McQueen-Mason, S., Farmer, T.J. & Gomez, L.D., Macromol. Rapid Commun. 2019, 40 (22), 1900361, Open Access
- Enzymatic synthesis of lignin derivable pyridine based polyesters for the substitution of petroleum derived plastics, Pellis, A., Comerford, J.W., Weinberger, S., Guebitz, G.M., Clark, J.H. & Farmer, T.J., Nature Communications 2019, 10, 1762, Open Access
- Recent Advances on Enzymatic Catalysis as a Powerful Tool for the Sustainable Synthesis of Bio-Based Polyesters, in Biorefinery: Integrated Sustainable Processes for Biomass Conversion to Biomaterials, Biofuels, and Fertilizers, Springer, 2019, 555-570
- Enzymatic synthesis of unsaturated polyesters: functionalization and reversibility of the aza-Michael addition of pendants, Pellis, A., Hanson, P-A, Comerford, J.W., Clark, J.H. & Farmer, T.J., Polym. Chem. 2019,10, 843-851
- Elucidating enzymatic polymerisations: Chain-length selectivity of Candida antarctica lipase B towards various aliphatic diols and dicarboxylic acid diesters, Pellis, A., Comerford, J.W., Maneffa, A.J., Sipponen, M.H., Clark, J.H. & Farmer, T.J., Euro. Polym. J. 2018, 106, 79-84, Open Access
- Efficient Physisorption of Candida Antarctica Lipase B on Polypropylene Beads and Application for Polyester Synthesis, Weinberger, S., Pellis, A., Comerford, J.W., Farmer, T.J. & Guebitz, G.M., Catalysts 2018, 8(9), 369, Open Access
- Effects of Methyl Branching on the Properties and Performance of Furandioate-Adipate Copolyesters of Bio-based Secondary Diols, Little, A., Pellis, A., Comerford, J. W., Naranjo-Valles, E., Hafezi, N., Mascal, M & Farmer, T. J., ACS Sustainable Chem. Eng. accepted, Open Access
- On the Effect of Microwave Energy on Lipase-Catalyzed Polycondensation Reactions, Pellis, A., Guebitz, G.M. & Farmer, T.J, Molecules 2016, 21, 1245, Open Access
Many platform molecules are either monomers themselves or are precursors to monomers. As such, we continue to work extensively in the development and application of new bio-based monomers and polymers. Our focus is primarily on exploiting the increased functionality of bio-based monomers and seeing how we can utilise this to prepare polymers with enhanced performance compared to petrochemicals.
We currently coordinate a large BBI-JU project, CHAMPION, developing new innovative circular bio-based polymers for use in home care additives, coatings and adhesives.
In collaboration with Biome Bioplastics, we have developed new polyesters to replace current petrochemical plastics such as PET and PBAT. These new materials utilised lignin-derivable pyridine diacid monomers.[1] The work was supported by the BBSRC/IUK through the EnzPoly project (BB/N023595/1). The study was recently extended to also consider pyridine diols.[2]
Figure 1: Lignin-derivable pyridine diacids as replacements for terephthalic and isophthalic acids.[1]
We have recently demonstrated that an innovative furan-derived photoreactive polyester can be formed via a combination of clean synthesis protocols to a furanbisacryl monomer followed by mild enzymatic polymerisation with suitable diols. Photoreactivity via [2+2] cycloaddition (supported by TD-DFT calculations) led to a cured material with enhanced tensile strength and work-hardening characteristics.[3]
Figure 2: Photocurable furan polyesters.[3]
In collaboration with Professor Mark Mascal at UC Davis, we developed a range of novel polyesters whose monomers are all derivable from single platform molecule, 5-(chloromethyl)furfuryl, where the inclusion of “methyl-branching” in the diols and diesters was found to enhance the properties of films formed.[4-6]
Figure 3: Novel methyl-branched polyesters from 5-(chloromethyl)furfural
Over several years, we have looked to exploit the chemicals functionality of itaconic acid in formation of polymers and are particularly interested in its unsaturated polyesters.[7] The lateral C=C of itaconate is ideal for post-polymerisation modification,[8] this allows the polymers final properties to be fine-tuned and enhanced, for example adding amine [9] or metal-chelating pendants.[10] Our studies have expanded understanding of known side reactions such as regioisomerisation to mesaconate or gelation via the Ordelt reaction,[11] and how they can be reduced.[12]
Figure 4: Synthesis of itaconate polyesters and subsequent post-polymerisation modifications via reactions of lateral methylene group.[10]
Further to this we have also shown how itaconate anhydride reacts directly with furfuryl alcohol (also bio-based) in a combined Diels-Alder addition and lactonisation to form an novel oxo-norbornene product. Remarkably the reaction is 100 per cent atom economic and can be carried out without solvent and the need for the catalyst (fig 5). Esterification of the carboxylic acid group yields monomers that can be polymerised via ring-opening metathesis polymerisation (ROMP).[13,14] Extending the study to maleic anhydride reacting with furfuryl amine allowed for monomers that can undergo more rapid ROMP.[15]
Figure 5: Novel ROMPable Oxa-norbornenes from the reaction of itaconic anhydride and furfuryl alcohol.[13]
We have also sought to develop new bio-based non-isocyanate polyurethanes (NIPUs) and have recently shown that a glucose-derived bis-cyclic carbonate can be combined with a lysine derived diamine to form self-foaming NIPU materials (fig 6).[16]
Figure 6: Wholly bio-based non-isocyanate polyurethanes (NIPUs).[16]
In collaboration with Leo Gomez (CNAP, Biology, York), we have developed a pathway from abundant pectin-rich waste biomass through to galactarate polyesters that furnished with multiple hydroxyl groups (fig 7).[17] The multidisciplinary approach required that application of both biological and chemical conversions and is an excellent example of how the two disciplines can work together to deliver highly functional bio-based polymers.
Figure 7: Galactarate polyesters. In collaboration with Leo Gomez and funded by the BBSRC LBNet grant ISCF05_Dec17 Gomez.[17]
The enzymatic synthesis of polyesters in solventless systems is an environmentally friendly and sustainable method for synthetizing bio-derived materials. Despite the greenness of the technique, in most cases only short oligoesters are obtained, with limited practical applications or requiring further chemical processing for their elongation. We recently presented a catalyst-free “thermal upgrade” of enzymatically synthesized oligoesters (adipates, isophthalates, furandioates and pyridinedioates), resulting in a 3-9 fold increase in Mw.[18]
Figure 8: Thermal upgrading of bio-based polyesters.[18]
Relevant Publications
-
- Enzymatic synthesis of lignin derivable pyridine based polyesters for the substitution of petroleum derived plastics, Pellis A., Comerford J. W., Weinberger S., Guebitz G. M., Clark J. H. & Farmer T. J., Nature Commun. 2019, 10, 1762. Open Access
- Enzymatic synthesis of biobased polyesters utilizing aromatic diols as the rigid component, Pellis A., Weinberger S., Gigli, M., Guebitz G. M. & Farmer T. J., Eur. Polym. J. 2020, 130, 109680, Open Access
- Work‐hardening photopolymer from renewable photoactive 3,3’‐(2,5‐furandiyl)bisacrylic acid, Lie, Y., Pellis, A., Funes-Ardoiz, I., Sampedro, D., Macquarrie, D.J. & Farmer, T.J., ChemSusChem 2020, 13, 4140-4150, Open Access
- Electrochemical Coupling of Biomass-derived Acids: New C8 Platforms for Renewable Polymers and Fuels, Wu, L., Mascal, M., Farmer, T. J., Perocheau Arnaud, S. & Chang, M. A. W., ChemSusChem 2017, 10, 166–17, Open Access
- New bio-based monomers: Tuneable polyester properties using branched diols from biomass, Perocheau Arnaud, S., Wu, L., Wong Chang, M-A., Comerford, J.W., Farmer, T.J., Schmid, M., Chang, F., Lib, Z.& Mascal, M., Faraday Discuss. 2017, 202, 61-77. Open Access
- Effects of Methyl Branching on the Properties and Performance of Furandioate-Adipate Copolyesters of Biobased Secondary Diols, Little, A., Pellis, A., Comerford, J. W., Naranjo-Valles, E., Hafezi, N., Mascal, M & Farmer, T. J., ACS Sustainable Chem. Eng. accepted, Open Access
- Synthesis of unsaturated polyester resins from various bio-derived platform molecules, Farmer, T. J., Castle, R. L., Clark, J. H. & Macquarrie, International Journal of Molecular Sciences 2015, 16, 14912-14932, Open Access
- Post-polymerization modification of bio-based polymers: Maximizing the high functionality of polymers derived from biomass, Farmer, T.J., Comerford, J.W., Pellis, A. & Robert, T., Polymer International 2018, (67), 775-789. Open Access via White Rose.
- Enzymatic synthesis of unsaturated polyesters: functionalization and reversibility of the aza-Michael addition of pendants, Pellis, A., Hanson, P-A, Comerford, J.W., Clark, J.H. & Farmer, T.J., Polym. Chem. 2019, 10, 843-851, Open Access vis White Rose
- Post-polymerisation modification of bio-derived unsaturated polyester resins via Michael additions of 1,3-dicarbonyls, Farmer, T. J., Clark, J. H., Macquarrie, D. J., Ogunjobi, J. K. & Castle, R. L., Polymer Chemistry 2016, 7, 1650-1658, Open Access via White Rose
- Insights into Post-polymerisation Modification of Bio-based Unsaturated Itaconate and Fumarate Polyesters via Aza-Michael Addition: Understanding the Effects of C=C Isomerisation, Farmer, T. J., MacQuarrie, D. J., Comerford, J. W., Pellis, A., & Clark, J. H, J. Polym. Sci. A 2018, 56(17), 1935-1945, Open Access
- Improving the post-polymerization modification of bio-based itaconate unsaturated polyesters: Catalyzing aza-Michael additions with reusable iodine on acidic alumina, Front. Chem. 2019, 7:501, Open Access
- Ring opening metathesis polymerisation of a new bio-derived monomer from itaconic anhydride and furfuryl alcohol, Bai, Y., De Bruyn, M., Clark, J. H., Dodson, J. R., Farmer, T. J., Honoré, M., Ingram, I. D. V., Naguib, M., Whitwood, A. C. & North, M., Green Chem. 2016, 18, 3945-3948, Open Access
- Wholly biomass derivable sustainable polymers by ring-opening metathesis polymerisation of monomers obtained from furfuryl alcohol and itaconic anhydride, Polym. Chem. 2017, 8, 3074-3081, Open Access via White Rose
- Rapid Ring‐Opening Metathesis Polymerization of Monomers Obtained from Biomass‐Derived Furfuryl Amines and Maleic Anhydride, Blanpain, A., Clark, J.H., Farmer, T.J., Guo, Y., Ingram, I.D.V., Kendrick, J.E., Lawrenson, S.B., North, M., Rodgers, G. and Whitwood, A.C., ChemSusChem 2019, 12(11), 2393-2401, Open Access via White Rose
- Renewable self-blowing non-isocyanate polyurethane foams from lysine and sorbitol, Clark, J. H., Farmer, T. J., Ingram, I. D. V., Lie, Y. & North, M., Eur. J. Org. Chem. 2018, 31, 4265-4271, Open Access via White Rose
- Sustainable Galactarate‐Based Polymers: Multi‐Enzymatic Production of Pectin‐Derived Polyesters, Vastano, M., Pellis, A., Botelho Machado, C., Simister, R., McQueen-Mason, S., Farmer, T.J. & Gomez, L.D., Macromol. Rapid Commun. 2019, 40 (22), 1900361, Open Access
- Thermal Upgrade of Enzymatically Synthesized Aliphatic and Aromatic Oligoesters, J.W., Comerford, Byrne, F.B., Weinberger, S., Farmer, T.J., Guebitz, G.M., Gardossi, L. & Pellis, A., Materials 2020, 13(2), 368, Open Access
Solvents are used in many processes throughout the chemical industry. At present, most are petroleum-derived, many are toxic and some cause damage to the atmosphere.
Using bio-based platform molecules and clean synthetic methodologies, such as flow chemistry, heterogeneous catalysis and enzymatic catalysis, new bio-based solvents can be designed and manufactured. These solvents can be subsequently used for the production of chemical intermediates, polymers and materials.
Figure 1. Synthesis of bio-based solvents, and other products, using bio-based solvents and clean synthetic methodologies from bio-based starting materials.
Current projects include ReSolve, funded by the Bio-Based Industries Joint Undertaking (BBI-JU) which operates under Horizon 2020. The ReSolve project aims to find replacements for toluene and N-methylpyrrolidone (NMP), two commonly used toxic solvents with different solvent properties.
Partners in this project, including Wageningen University (WUR), Bio-Based Europe Pilot Plant (BBEPP), Nitto Belgium, Circa, Norske Skog, Avantium, Process Design Centre (PDC), TNO, BioDetection Systems (BDS) and Nova Institute, provide expertise in a wide range of areas vital for solvent development.
In other work, a family of n-butyl diamides from succinic acid were designed to eliminate toxicity while maintaining high polarity (fig 2). This was moderately successful; no alarming indications of CMRS activity were observed and polarity was high, albeit not as high as predicted. The solvents could be synthesised using K60 silica as a catalyst, and were found to be useful in a range of applications - Heck reaction, MOF synthesis and membrane preparation.
Figure 2. Hypothesized effect on polarity and toxicity of having two n-butyl-amide groups on one molecule.
Some ethers, esters and ketones have been identified as potential replacements for toluene in many applications, in particular in radically-initiated polymerizations. The esters and ketones were less polar than ethyl acetate, but possessed boiling points no higher than that of toluene. Two of these, TMO and pinacolone, were found to be useful for enzymatic polymerization of adipate and C4, C6 and C8 primary diols. Compared to toluene, TMO and pinacolone could achieve higher conversion and Mw and at lower temperatures (fig 3).
Figure 3. Enzymatic synthesis of poly(1,4-butylene adipate) using Candida antarctica lipase B immobilized via adsorption onto polyacrylate beads at 30 °C, 50 °C and 85 °C.
Recent Publications
- Optimization of amidation reactions using predictive tools for the replacement of regulated solvents with safer biobased alternatives, Petchey, T.H.M., Comerford, J.W., Farmer, T.J., Macquarrie, D.J., Sherwood, J. & Clark, J.H., ACS Sustainable Chem. Eng., 2017, 6, 1550-1554.
- 2,2,5,5-Tetramethyltetrahydrofuran (TMTHF): a non-polar, non-peroxide forming ether replacement for hazardous hydrocarbon solvents, Byrne, F., Forier, B., Bossaert, G., Hoebers, C., Farmer, T.J., Clark, J.H. & Hunt, A.J., Green Chem., 2018, 19, 3671-3678.
- Challenges in the development of bio-based solvents: a case study on methyl (2, 2-dimethyl-1, 3-dioxolan-4-yl) methyl carbonate as an alternative aprotic solvent, Jin, S., Byrne, F., McElroy, C.R., Sherwood, J., Clark, J.H. & Hunt, A.J., Faraday Discussions, 2017, 202, 157-173.
- Solvents from waste, Byrne, F., Jin, S., Sherwood, J., McElroy, C.R., Farmer, T.J., Clark, J.H. & Hunt, A.J., Bio-Based Solvents, John Wiley & Sons, Ltd, 2017, pp. 49–82.
- Tools and techniques for solvent selection: green solvent selection guides, Byrne, F.P, Jin, S., Paggiola, G., Petchey, T.H.M., Clark, J.H., Farmer, T.J., Hunt, A.J., McElroy, C.R. & Sherwood, J., Sustainable Chemical Processes, 2016, 4(7).
- Rapid and efficient biphasic liquid extraction of metals with bio-derived lipophilic β-diketone, Asemave, K., Byrne, F., Farmer, T.J., Clark, J.H., Hunt, A.J., RSC Advances, 2016, 6, 95789-95792.
- A Family of Water Immiscible, Dipolar Aprotic, Diamide Solvents from Succinic Acid, FP Byrne, CM Nussbaumer, EJ Savin, RA Milescu, CR McElroy, JH Clark, BMA van Vugt-Lussenburg, B van der Burg, MY Meima, HE Buist, D Kroese, AJ Hunt, TJ Farmer, ChemSusChem, 2020, 13, 3212-3221.
- Fabrication of PES/PVP Water Filtration Membranes Using Cyrene®, a Safer Bio-Based Polar Aprotic Solvent, RA Milescu, CR McElroy, TJ Farmer, PM Williams, MJ Walters, and JH Clark, Advances in Polymer Technology, 2019, 9692859.
Patents
- US 10738021 B2 – Preparation of TMTHF
- EP 3500561 B1 – Preparation of TMTHF
- JP 6633257 B2 – Preparation of TMTHF
- CN 109790134 A – Preparation of TMTHF
Contact us
Green Chemistry Centre of Excellence
greenchemistry
+44 (0)1904 322567
Department of Chemistry,
University of York,
Heslington,
York YO10 5DD,
United Kingdom.
@@GreenChemYork
Facebook